Adenovirus Vaccine Manufacturing Process
What are Adenoviruses
Adenoviruses are non-enveloped viruses with a ds-DNA, 70–90 nm in size. They are efficient at transducing target cells and can be produced at high titers (>1011/ mL). Adenovirus (AV) in its normal form is a pathogen that causes respiratory ailments such as conjunctivitis and the common cold. It is also capable of infecting neurons, damaging cells, and invoking strong immune responses. However, adenoviruses used as vectors are specially designed not to cause any disease and are generally regarded as safe.
Overcomes Pre-existing Immunity Towards Adenoviral Vectors Using Simian-derived Vectors
Although adenoviral vectors elicit both innate and adaptive immune responses, a major drawback of these vectors is the pre-existing immunity towards them. More than fifty serotypes of adenovirus infect humans and more than 80% of the population has been exposed to at least one serotype and developed a serotype-specific immune response. This preexisting immunity against the vector can significantly reduce immune response to the vector’s payload (the pathogen antigen expressed from the transgene inserted in the vector).
In recent years, adenovirus vaccine development has explored use of simian-derived adenoviral vectors, which have negligible seroprevalence in the human population. While overcoming the problem of pre-existing anti-vector immunity, they retain the advantages of other replication-incompetent adenoviruses:
- Induction of a broad cellular and humoral immune response against the target antigen
- Safety, with no viral replication in the vaccine recipient due to deletion of essential genes
- Lack of host genome integration
- Efficient infection of a variety of mammalian cell types including antigen-presenting cells
- Compatibility with different processing technologies including stirred tank bioreactors, high-capacity filtration methods and chromatographic purification procedures as a result of their neutral charge and relatively small size. This size (c 80-90 nm) is compatible with final sterile filtration (0.2 µm) eliminating the need for a validated and cumbersome closed processing approach.
See our case study on the development of a rapid, scalable, and GMP-compliant manufacturing process for simian adenoviral vector vaccines
The Adenovirus-based Viral Vaccine Manufacturing Process
The manufacturing process for adenovirus vectors is straightforward and fairly templated; a general outline is shown in Figure 1. Typical pilot scale bioreactor size for adenovirus production is 20 L cell culture, which becomes ~4 L after UF/DF and ~500 mL post chromatography and final sterile filtration. Full-scale process volumes range from 100 to 200 L, and overall process yield is typically 65%.

Figure 1.Generic adenovirus-based vectored vaccine process. A: Media and Inoculum Preparation B: Cell Growth in Bioreactor Virus Inoculation C: Clarification D: Nuclease Treatment E: Ultrafiltration/Diafiltration F: Virus Purification G: Ultrafiltration/Diafiltration H: Final Filtration
Cell Culture for Producing Adenovirus Vectors
Efficient manufacturing of adenovirus vectors can be accomplished using genetically engineered human cell lines that complement the deleted adenoviral genes required for replication (e.g., 293-ORF6 cells, HEK293, PER.C6). These cells have a well-characterized safety profile and can be adapted for growth in serum-free suspension. Production cells are grown in stirred-tank bioreactors with serum-free culture medium.
During the adenovirus infection phase, the metabolic processes of the production cell line are significantly increased to support vector manufacture. In order to facilitate successful vector production, a medium exchange step is performed to remove spent medium containing metabolites such as lactic acid, which can be detrimental to virus production. The virus yield drops significantly when the media pH <7. A 50–100% typical cell density during adenovirus infection is 0.5–9.0 x 106 cells/mL. The adenovirus titer during harvest generally ranges from 109 to 1011 pfu/mL.
Cell Lysis and Clarification to Harvest Adenovirus
Cells are lysed either mechanically or by a chemical lysis agent (e.g., non-ionic detergent) for the harvesting of adenoviruses. Lysis with Triton™ X-100 solution is most common. Clarification is performed to remove the cells or cell debris and harvest adenoviruses. A depth filter is commonly used for primary clarification. Some manufacturers also use tangential flow filtration (TFF) at low shear conditions depth filtration or normal flow filtration (NFF) for clarification of adenoviral vaccine harvests. Filter capacities depend on cell density at harvest, the degree of lysis, and the particle size distribution. Typical lysate turbidity is >200 NTU. Similar to the viral vaccine process, depth filter followed by bioburden reduction filter is commonly used for this application. TFF may also be an option in addition to the NFF options. Typical loading for TFF is 20-30 L/m2. Some manufacturers also use centrifugation for primary clarification. Filtrate from secondary clarification has been, in some instances, filtered through 0.45 µm for bioburden reduction (~250–500 L/m²). Post clarification turbidity is in the range of 5–10 NTU. This unit operation is conducted at room temperature.
Nuclease Treatment to Remove Contaminating Nucleic Acids
Carryover nucleic acid from lysed cells is a key contaminant in adenovirus vaccine processes and can interfere with virus purification. Viruses propagated in non-human cells (i.e., HEK293, PER.C6) pose a greater risk of nucleic acid carryover. Regulations require that carryover host cell nucleic acid content should be below 10 ng/dose of attenuated viral vaccine. Virus harvest is treated with about 0.9-1.1 U/mL of Benzonase® endonuclease at 30-34 °C for four to eight hours.
Ultrafiltration/Diafiltration
After Benzonase® endonuclease treatment, the harvest is diafiltered using TFF (100-300 kDa UF devices). The typical flux for 300 kDa is ~25–50 LMH at 5–10 psi TMP at 5–7 L/min/m2 feed flow rate. Next, 4–10 X concentration and 5–8 N diafiltration are performed. More than 99% retention of adenoviruses is typical. Some manufacturers perform a vector concentration step to reduce overall volume before Benzonase® endonuclease treatment. Diafiltration is then performed to facilitate buffer exchange for further processing, such as downstream chromatographic processing. Sometimes an overnight hold step is employed prior to downstream purification. Consequently, a filtration step is performed to reduce the risk of bioburden and to protect the downstream chromatography columns.
Chromatography Methods for Adenovirus Purification
Small-scale clinical lots are typically purified using CsCl-based density gradient ultracentrifugation. However, for large-scale production, column chromatography is employed. Two- or three-step column chromatography purification is normally used for adenovirus production. Purification methods commonly used are ion exchange and size exclusion chromatography (optional). Anion exchange is used to remove HCP, DNA, RNA, and other major contaminants. Size exclusion chromatography is used for trace contaminant removal. Typically, in anion-exchange columns, the adenovirus feed (5 × 1012 virus particles/mL of resin) is loaded at 75 cm/hour flowrate in 50 mM Tris-HCl, pH 8.0 in 5% glycerol and eluted in salt gradient. Adenovirus elutes at ionic strength of 40 mS/cm. Weak ion-exchangers are also proven to work for purification of adenovirus resulting in high purity and yield.
Sterile Filtration
Sterile filtration ensures the sterility of the final formulated product. A filter pore size of 0.22 µm or less is required to eliminate microbial contaminants.
Case Study: Developing an Accelerated and More Cost-Effective Single-Use Adenoviral Vector Vaccine Manufacturing Process through Public-Private Collaboration
In this case study, we collaborated with the Jenner Institute, Oxford University in the United Kingdom to develop an optimized, single-use GMP process for manufacturing simian adenoviral vector-based vaccines including those targeting common pathogens such as rabies and emerging threats like Zika and Ebola. Our goal was to improve the manufacturing process for adenoviral-based vaccines for Phase 1 materials at a scale of >5x1013 VP (virus particles; equivalent to 2000 doses) which could then be readily scalable to 5x1014 VP. We sought to develop a cost-effective and transferable template with a minimum of 50% overall efficiency that could be used to accelerate vaccine development and manufacturing worldwide. The first candidate evaluated as a pilot was the rabies vaccine.
A more robust template for adenoviral vector manufacturing based on an easy-to-operate and easy-to-implement GMP single-use process has the potential to deliver a number of benefits including:
- Accelerating clinical phases
- Reducing time to market
- Enabling a more rapid response to outbreaks and pandemics
- Meeting the need for more affordable medicines in low resource settings
The transition to a new manufacturing template focused on reducing the need for handling and compressing processes in order to evolve away from labor intensive, risk-associated operations. We sought to eliminate shake flasks which must be opened in a manual process, centrifugation because of the associated maintenance, investment and the scalability challenges and ultra-centrifugation.
The new template compressed the process into seven steps and maximizes use of disposable technologies. In addition, we aimed at developing a template that can be used to manufacture different adenoviral vectors and tested two other candidates in parallel to the rabies vaccine.
Upstream Processing with Single-use Bioreactors
For the new upstream process, Mobius® three-liter and other single use bioreactors were run in fed-batch mode after inoculation with a seed train of HEK293 T-rex. A significant increase in yield was achieved by converting from shake flasks to single use bioreactors. Upstream process yields per bioreactor vessel ranged from 7.2x1013 to 2.5x1014 VP (qPCR) across one run with ChAdOx2 RabG, two runs with ChAdOx1 RVF, and two runs with ChAd63 ME-TRAP, i.e. >2500 human doses of c. 2.5x1010 VP.
DNA Reduction
Nucleic acid digestion to meet regulatory requirements and facilitate downstream processes was accomplished using Benzonase® nuclease. Addition of the nuclease at 60 U/mL of cell culture media decreased the level of host cell DNA >1500 fold during the two-hour lysis in the bioreactor, meeting the regulatory requirement of < 10ng/dose. The overall process was compressed as the lysis was performed directly in the bioreactor. As shown in Table 1, lysis was sufficient after only 30 minutes, reaching the target of 10ng/dose; after two hours, the reduction is more than 1,500-fold. In the light of the results, it is likely that a lower concentration would suffice to reach the 10 ng/ dose and DNA digestion < 200 base pair requirement. Further optimization could be performed to achieve sufficient DNA digestion within a satisfactory time using a dose of Benzonase® nuclease more suitable for scalability.
Removal of DNA and its impact on viscosity also reduced the potential for blockages and the need for a larger filtration area in downstream operations.
Replacing Centrifugation in Downstream Processing with Disposable Depth Filters
For this new process, we replaced the centrifugation unit operation with clarification using depth filters which are disposable and used in normal flow filtration and thus easy to operate. Because clarification has a strong impact on subsequent downstream steps, we sought to achieve as high a recovery as possible, in this case, greater than 90% along with turbidity reduction.
While adenoviral vectors are neutral, we still wanted to test different filters and select the best option to maximize adenovirus recovery. Table 2 describes the three Millistak+® depth filters and two Clarisolve® depth filters that were tested; each were different grades, micron ratings and charge.
Figure 2 summarizes our evaluation of the different filters listed in Table 2. Virus recovery was similar for all and achieved our goal of >90% as measured by qPCR. The high capacity Millistak+® HC Pro COSP filter performed best in terms of turbidity reduction throughput; we achieved a single-step turbidity reduction from 70 to 7 NTU. Additionally, we were able to process more than 2.3L without reaching a true final end pressure point (Pmax). In the small-scale trials, we actually never reached a true Pmax, which is a constant flux methodology with pressure monitoring. The solution then went through a bioburden reduction step using an Opticap® XL 150 Millipore Express® 0.2 micron filter before moving to purification.
To remove the requirement for ultracentrifugation at this point, we opted for tangential flow filtration. We achieved an 80-88% product recovery and 20-30x reduction in host cell protein contaminants using the following method:
- 300 kDa Pellicon® 2 Mini filter (0.1m2) with Biomax® membrane
- 5L starting volume after clarification (Millistak+® 10x volume concentration)
- 10 DV filtration against IEX-suitable buff er (containing 100 mM NaCl)
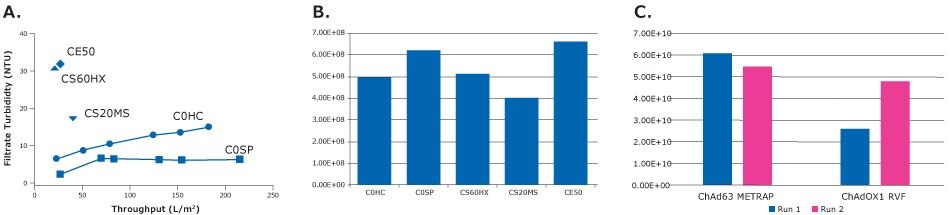
Figure 2.Evaluation of virus recovery using different depth filters. The Millistak+® HC Pro COSP performed best in terms of turbidity reduction and virus recovery. A: Throughput (L/m2) B: chAdOx2-RabG – Titers after primary clarification VP/mL (smale scale trials) C: Virus titers after clarification with Millistak+® HC Pro C0SP (VP/mL) (2-4 L scale)
A key factor when performing tangential flow filtration, and something we recommend when handling viruses with ‘open’ membranes (>100 kDa MWCO, potentially permitting high flux and high conversion fractions even with low transmembrane pressure (TMP), is permeate control. A permeate control is performed with addition of a pump or valve to partially restrict the permeate site to avoid the creation of an uncontrolled polarization layer. Once the TMP is stable, less stress is applied to the viruses and this helps ensure better recovery and reduce fouling (Figure 3).
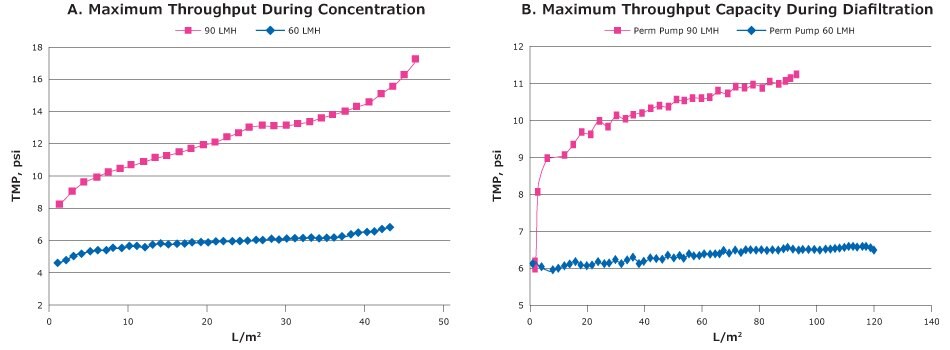
Figure 3.Small scale trials of the Tangential flow filtration experiment with clarified lysate of ChadOx2-RabG using 300 kDa MWCO Biomax® PESC-screen Pellicon® 2 Mini cassette. A: TMP excursion during concentration B: TMP excursion during diafiltration.
The objective of the chromatography step was to use disposable membranes instead of resins which must be packed, cleaned and validated. To this end, we leveraged our Natrix® Q chromatography membrane, a quaternary amine grafted on a macroporous hydrogel-coated polypropylene membrane. This design combines the advantages of traditional resins and absorptive membranes leading to high-capacity and fast flow rates, allowing us to further compress the process and reduce costs. This technology is highly versatile and can be used in flow through or bind-elute modes, with ion exchange and affinity methods. For this process, we evaluated the anionic exchange version and performed the chromatography in a bind-elute mode using the parameters in Table 3.
A good elution of the rabies vaccine candidate was obtained with Natrix® HD-Q membrane adsorber in bind & elute mode when loading in a phosphate based buff er (Figure 4 & 5). With the membrane, we were able to achieve a DBC of 6, an 85% reduction in host cell protein along with a satisfactory 76% recovery of the virus. DBC was defined as the load at which breakthrough A280 exceeded that observed early during loading (i.e. flowthrough A280) by 10% of the difference between the A280 of the loaded sample and the flowthrough A280.
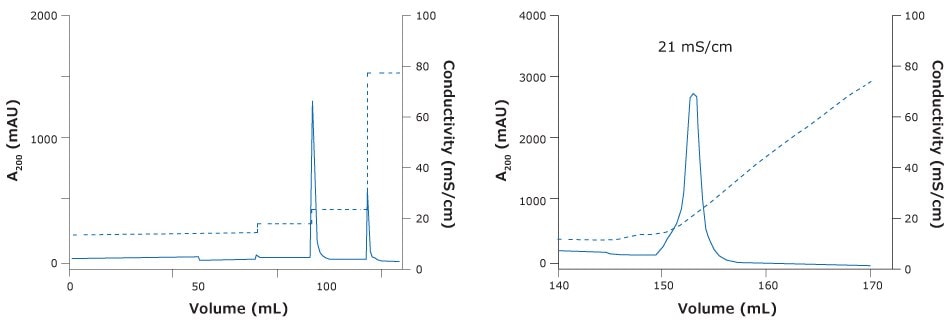
Figure 4.Chromatography of ChAdOx-2-RabG vaccine using Natrix® HD-Q membrane adsorber. (Left) Step elution of ChAdOx2-RabG with NatrixHD Q membrane adsorber. (Right) Gradient elution ChAdOx2-RabG elution peak at 21 mS/cm.
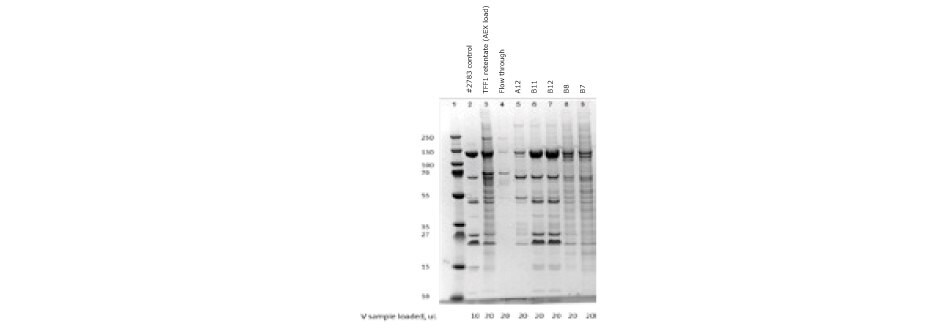
Figure 5.SDS-PAGE of ChAdOx-2-RabG vaccine after anion exchange chromatography. Elution peak samples were loaded in lines B11 and B12.
Single use flowpath
A further objective of the work was to rely on single-use technologies in the development of the manufacturing platform. pH & DO control can be achieved on the single use Mobius® 3 L bioreactors using commercially available invasive single use probes. The upstream process can be scaled up to a 2000 L Mobius® single use bioreactor, which includes a single use bag with an external loop dedicated to sampling either with multiuse or single use probes. Rigs for clarification & TFF were designed to ensure a closed process.
Performance Summary
Our collaboration with the Jenner Institute has resulted in a single-use, scalable GMP template for manufacture of adenoviral-based vaccines. The new approach compresses the time required to manufacturing doses to two days for upstream operations plus clarification and DNA digestion, followed by three days for the purification, which is solely based on filtration. This is critically important during an outbreak.
The new process is a full single-use flow path including bioreactors, tubing and liners, collection bags, filters and chromatography membranes. We met our initial goals of producing at least 2000 doses from a 4L culture with the necessary host cell protein removal and virus recovery. This process can be scaled to 2000L, using different single-use technologies such as bioreactor bags and mixing systems for media and buffer storage and intermediate mixing and storage.
This successful collaboration is an example of how public and private organizations can come together to solve challenges facing our industry and deliver meaningful solutions to benefit the global population. With this faster and more cost-effective approach to vaccine manufacturing, we take an important step towards expanding access, address shortages in supply and accelerate our response to outbreaks and pandemics.
Summary
Viral vectors are vehicles that deliver the genetic payload to target cells. Advancements have been made in the vector design to ensure safety of these types of vaccines. Compared to the conventional vaccines, adenovirus vector-based vaccines can express a wide range of antigens from virus, bacteria, or protozoan. They elicit long-term immune responses against infectious diseases.
Related Products
References
To continue reading please sign in or create an account.
Don't Have An Account?