Sialic Acid
Sialic acid is a critical part of glycan structures. Uncover the functions of sialic acid, its synthesis, modifications, signaling, and more. Also, find products for sialic acid research conveniently separated by categories.
Read more about
What Is Sialic Acid?
Sialic acids are classified as carbohydrates, but they are structurally unlike any of the other common sugars. Although they do not contain the strict polyol structure ([-CHOH-]n) typical of core carbohydrates, sialic acids are substituents of glycan structures, appearing most frequently as the non-reducing terminal molecules of N-glycans, O-glycans, and glycosylphosphatidylinositol (GPI) anchored proteins. Sialic acids also differ from other sugars in that they are less commonly utilized as an energy source but are critical to development, cellular recognition, cell-cell attachment, and signaling. All eukaryotic systems and several prokaryotes express sialic acids, and other pathogenic bacteria, viruses and parasites utilize cell surface sialic acids as ligands as a means to adhere to cells, with the influenza viruses being the most well-known example of sialic-binding pathogens.
Sialic Acid Synthesis and Signaling
All sialic acids are based on a cyclic nine-carbon structure with a carboxylic acid group at the C1 position (see Figure 1). Because of the carboxylic acid group, sialic acids have an inherent negative charge. The most common sialic acids are N-acetylneuraminic acid (Neu5Ac, NeuNAc or NANA), N-glycolylneuraminic acid (Neu5Glc), and N-acetyl-9-O-acetylneuraminic acid (Neu5,9Ac2).1 Substitutions may be made at carbons C4, C7, C8, and C9 and the amine located at C5, and multiple substitutions contribute to the variety of possible structures. The hydroxyl groups located at C4, C7, C8, and C9 may undergo acetylation, phosphorylation, sulfation, or methylation. Over 50 forms of sialic acid have been identified to date, due to the number of locations available for structural modification as well as core variation. An additional factor that contributes to sialic acid complexity in vivo is the migration of an O-acetyl ester moiety between C7 and C9 under certain physiological conditions.
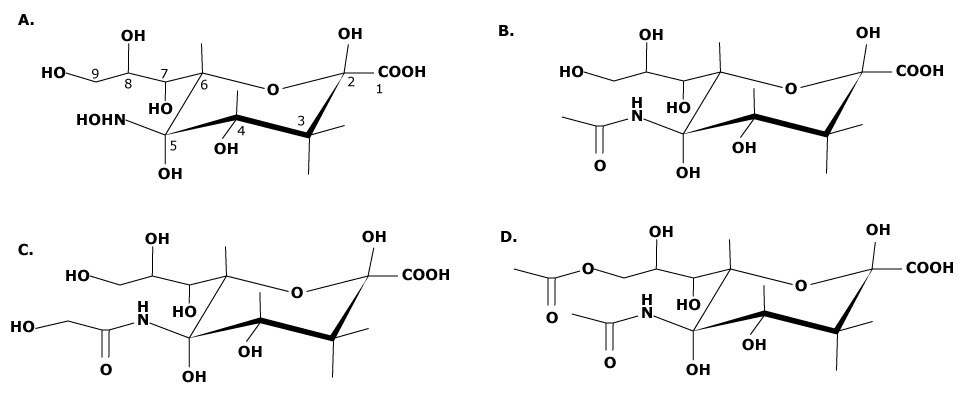
Figure 1.(a) Neuraminic acid, the core structure of all sialic acids. Structural modifications can be made at C4, C7, C8, C9, and at the amine group of C5. (b) Structure of N-acetylneuraminic acid (Neu5Ac). (c) Structure of N-glycolylneuraminic acid (Neu5Gc). (d) Structure of N-acetyl-9-O-acetylneuraminic acid (Neu5,9Ac2).
The life cycle of vertebrate sialic acids follows a clear pathway, moving from synthesis to transport, attachment, and removal. After removal, the sialic acids may be recycled by attachment to cytidine 5'-triphosphate (CTP) for reuse as a substrate, or the sialic acid may be degraded by the action of sialic acid-specific pyruvate lyase and esterases (see Figure 2). Altheide, et al., outlined the processes in which sialic acids participate into five steps2:
- Biosynthesis – the construction of sialic acids from precursors
- Activation, transport and transfer – the generation of sialic acid donor substrates and attachment to glycans via the action of transferases
- Modification – the addition and relocation of modifications to the sialic acid
- Recognition – the binding or sialic acid recognizing proteins to sialoglycans
- Recycling and degradation – the process of enzymatically removing sialic acid residues and degrading free sialic acid.
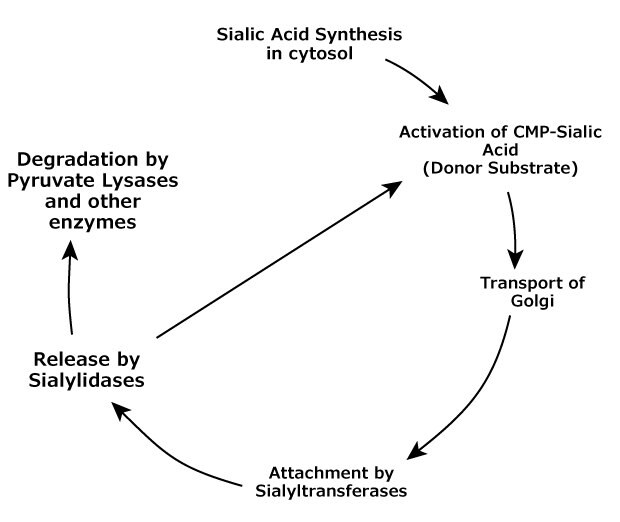
Figure 2.Sialic acid metabolic lifecycle in vertebrates.
Biosynthesis of Sialic Acids
Sialic acid synthesis in animals takes place primarily in the cytosol and involves four enzymes participating in a five-step process (see Figure 3). The dual activity enzyme UDP-GlcNAc 2-epimerase/ ManNAc-6-kinase (gene symbol GNE) converts the starting substrate uridine 5'-diphosphate-N-acetyl-D-glucosamine (UDPGlcNAc) to N-acetyl-D-mannosamine (ManNAc) with removal of the UDP moiety and epimerization of the carbohydrate. The kinase function of the same enzyme phosphorylates the sugar to produce N-acetyl-d-mannose 6-phosphate (ManNAc-6-P). The condensation reaction between phosphoenol pyruvate (PEP) and ManNAc-6-P initiated by NeuAc-9-P-synthetase (gene symbol NANS) results in the phosphorylated sialic acid precursor N-acetylneuraminic acid 9-phopshate (Neu5Ac-9-P). This precursor is dephosphorylated by Neu5Ac-9-P-phosphatase (gene symbol NANP) to produce the key sialic acid N-acetylneuraminic acid (2-ketoacetamido-3,5-dideoxy-D-glycero-D-galactononulosonic acid; Neu5Ac).
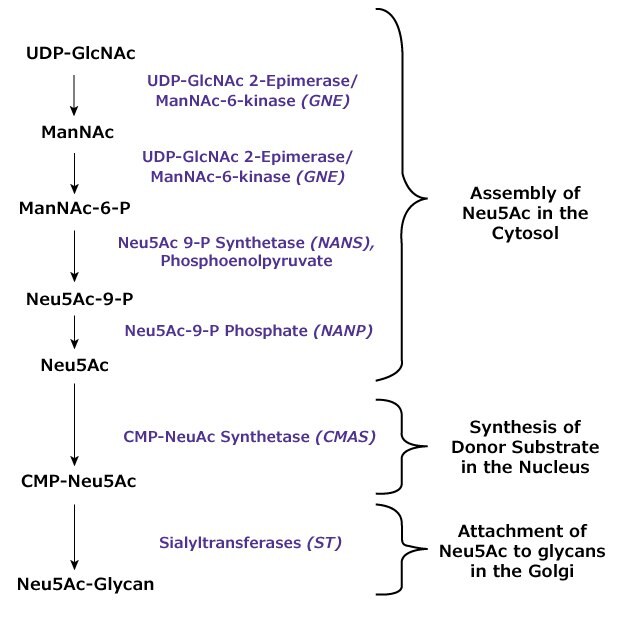
Figure 3.Schematic of N-acetylneuraminic acid (Neu5Ac) enzymatic synthesis and attachment to glycan structures.
Transfer of Sialic Acids
After biosynthesis, Neu5Ac must be incorporated as a nucleotide donor substrate for subsequent transfer to an oligosaccharide structure. This substrate creation takes place in the nucleus of eukaryotic cells. Neu5Ac is transported to the nucleus; whereupon, it is attached to cytidine 5'-triphosphate (CTP) to produce the donor substrate CMP-Neu5Ac with the loss of pyrophosphate via catalysis by the enzyme CMP-NeuAc synthetase (gene codeCMAS). The CMP-Neu5Ac substrate is then transported back to the cytoplasm and then to the Golgi for use by sialyltransferases.
The sialyltransferases (ST) of the Golgi attach Neu5Ac residues to oligosaccharides using CMP-Neu5Ac as the sugar donor. These transferases produce specific glycosidic linkages for sialic acid (α2→3, α2→6, or α2→8) and have preferences for monosaccharide acceptors used in attachment. These specificities are indicated by the enzyme nomenclature, e.g., ST6Gal I is a sialyltransferase that forms α2→6 linkages to galactose.
Enzyme inhibitors are commonly designed based on structural similarity to functional receptors or substrates, with the expectation that the inhibitor will be incompletely processed by the enzyme and the reaction will terminate abnormally. This concept was used in experiments using ManNAc analogs containing unnatural structures as a way to prevent sialic acid synthesis. However, instead of interfering with sialic acid synthesis, the analogs were processed through enzymatic synthesis with their unnatural component intact and subsequently incorporated at the terminus of oligosaccharides. This lack of substrate selectivity may not be completely surprising as it has been shown the sialic acid synthesis pathway is necessary for vertebrate survival. Elimination of UDP-GlcNAc-2-epimerase was found to be lethal in a knockout mouse model.3The ability of unnatural sialic acid analogs to be incorporated into glycan oligosaccharides has allowed researchers to use this substrate flexibility as a means for study of sialylation contribution to cell biology. Metabolic incorporation has been combined with chemoselective ligation strategies, in which the unnatural group of the sialic acid analog is available for covalent bonding to a detection or attachment molecule.4Campbell, et al., reviewed the unnatural monosaccharides that could be incorporated through metabolic labeling, withN-acetyl-D-mannosamine and sialic acid analogs being the most successful for use in oligosaccharide engineering.5Sialic acid metabolic engineering has also been used in the study of viral adhesion and innate immune system regulation. Unnatural mannosamine analogs have been used to investigate the glycan specificity requirements of viral hemagglutinin binding and the immunotargeting of cancer cells that express polysialic acid.5,6
Modification of Sialic Acids
Modification to the neuraminic acid takes place in the Golgi, either before the carbohydrate moiety is transferred to a carbohydrate acceptor or after transfer. The exception to this is the development of N-glycolylneuraminic acid (Neu5Gc), the hydroxylated form of Neu5Ac. In non-human vertebrates, including other primates, Neu5Ac is converted to Neu5Gc in the cytosol by the action of cytidine monophosphate-N-acetylneuraminic acid hydroxylase (CMP-N-acetylneuraminate monooxygenase; gene symbol CMAH). Human evolution has resulted in the elimination of this gene, so while Neu5Gc is a common sialic acid in other vertebrates, it does not occur naturally in man.2 The enzymes and mechanisms by which sialic acids are subsequently modified are not well defined.
Sialic Acid Recognition and Biological Function
Sialic acids and sialylated glycoproteins and glycoconjugates are necessary for proper mammalian development. Because sialic acids are typically located at the terminal end of the glycan structure and because of their acidic, negatively charged nature, sialylated glycoconjugates can inhibit many intermolecular and intercellular reactions. As cited previously, the ability to synthesize sialic acids was shown to be necessary for murine survival and development.3 Polysialic acid is a post-translational modification of the neural cell adhesion molecule (NCAM) glycoprotein and its presence is necessary for postnatal neuron development, while sialic acid is a critical component of brain gangliosides (sialylated glycosphingolipids), and dietary sialic acids are necessary for normal mammalian brain development.7
Sialic acids are a critical part of the innate immune system in vertebrates. Key proteins that recognize sialic acids are the I-type lectins that include the major subfamily of sialic acid binding immunoglobulin-like lectins (Siglecs). Briefly, Siglec proteins are expressed on the cell surface and are highly specific for sialylated ligands. Terminal sialic acids on cell surface glycoconjugates act as ligands for cell surface Siglecs, masking the Siglecs and preventing binding to external pathogens. A discussion of Siglecs and their roles in the innate immune system is not included here; the reader is directed to definitive reviews for further information.8-10 By attaching to nearby sialylated glycans also on the cell surface, these proteins produce "cis" bonds that reduce the likelihood of viral attachment and help to protect ("mask") the sialylated glycans from external attachments to viral hemagglutinins.
This cis binding of terminal sialic acids to Siglecs has been described as identifying the cell as "self" to the immune system and preventing attack by macrophages and other cells of the immune system.8 Loss of terminal sialic acids through sialidase cleavage unmasks the Siglecs and makes the Siglecs available for binding to another host cell ("trans" binding) or to a pathogen that has stronger affinity for the Siglec binding site. Terminal sialic acids also protect penultimate glycoconjugate sugars, primarily galactose, from carbohydrate binding receptors such as galectins (vertebrate galactose-specific lectins) that are associated with inflammation, apoptosis, and immune cell responses.
Another set of membrane proteins that has affinity for sialylated ligands is the selectins, a family of calcium-dependent lectins expressed by endothelial cells, leukocytes, and platelets. The selectins have a weak affinity for the sialylated Lewis X antigen (SLeX) that has been associated with cancer, and many of the identified ligands with higher affinity for selectins are both sialylated and fucosylated. Selectins expressed on the surface of vascular endothelial cells in areas of inflammation adhere to sialylated ligands present on leukocytes and platelets. This weak adhesion tethers the circulating leukocytes and slows their transport through the venule. The leukocytes are tethered briefly and roll along the endothelial cell surface, slowing their transport and allowing signaling by chemokine receptors to activate integrin expression by the leukocytes. The leukocytes are finally attached to the vascular surface through integrin-immunglobulin superfamily binding and migrate through the vascular wall to the damaged tissue.
N-Acetylneuraminic acid has been shown to be able to scavenge free radicals including hydrogen peroxide and lipid hydroperoxides,11 and other sialic acids may also have antioxidative activities. Bovine submaxillary mucin was able to reduce hydroxyl radical degradation of plasmid DNA, but desialylated mucin did not prevent DNA oxidative degradation.12 Treatment with intravenous sialic acid was shown to counteract lipopolysaccharide induced liver toxemia in rats.13
Sialic acid is a component of the α-subunit of voltage-gated sodium channels, in which the subunit molecule contains an estimated 100 sialic acid residues. Insufficient sialylation of the subunit, either when expressed in a cell line unable to properly sialylate proteins or by deglycosylation, results in channel gating that is more depolarized. Sialylation of the β1-subunit has been shown to indirectly support sodium channel gating by the α-subunit.14 This sodium channel gating function of sialic acid has been examined as a possible target for the treatment of epileptic seizures. Treatment of rat hippocampus with neuraminidase to reduce the number of surface sialic acid residues found that both in vitro and in vivo models of epilepsy demonstrated reduced seizure susceptibility.15
Changes in sialylation expression, including increases in level of sialylation and changes to the sialic acid modifications have been identified in cancer cells. The increase in sialylation in cancer may be due to overexpression of sialyltransferases.16 Sialylated Lewis X (SLex)and sialylated Lewis a (SLea) (see Figure 4) carbohydrate structures were identified as tumor antigens with higher levels of expression in several types of cancer cells.17 SLex acts as a ligand for endothelial E-selectin , a selectin that is expressed after induction by inflammatory events in a cytokine-dependant manner. Sialyl Lewisx/a may play a role in E-selectin mediated cancer cell adhesion to the endometrium.18 Increases in serum sialic acid levels have also been found in patients with diabetes or hypertension.19
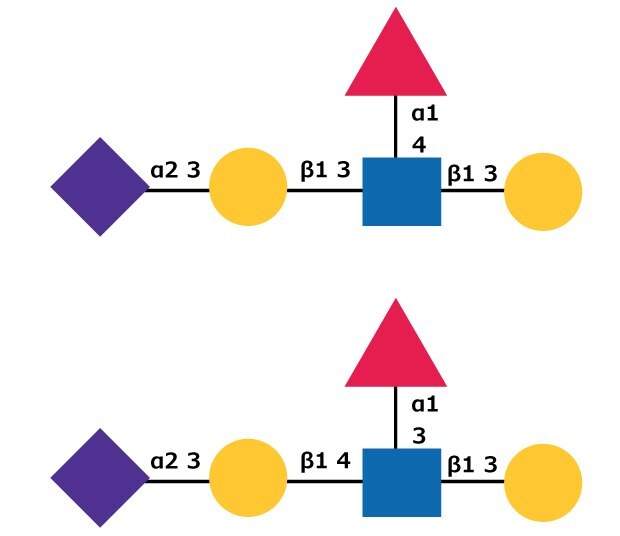
Figure 4.Carbohydrate structures for sialyl Lewisa (top) and sialyl Lewisx (bottom) oligosaccharides. See common symbol nomenclature to identify carbohydrates.
Sialic Acids and Pathogens
Several pathogenic organisms including the influenza virus exploit cell surface sialic acids for attachment and infection.20 Influenza has two complementary activities toward cell surface sialic acids, a hemagglutinin activity that has binding preference for sialic acids and a neuraminidase activity that is able to cleave terminal sialic acid residues from oligosaccharides. The common abbreviation for influenza strains reflects the presence of hemagglutinin (H) and neuraminidase (N). Viruses use the eukaryotic surface terminal sialic acids as ligands to attach to a cell for infection. These "trans" attachments typically have a low binding coefficient, so in order to strengthen the bonds to the host cell, there are typically a large number of attachments between the virus and the host. The binding allows the virus to transfer its RNA to the host cell for subsequent infection and copying. There are 16 known serotypes of influenza hemagglutinins, and these have specific receptor preferences for terminal sialic acids. Avian viral hemagglutin preferentially binds to sialic acids linked α(2→3) to galactose, while human viral hemagglutinins have greater affinity for sialic acids with α(2→6) linkages.21,22 This linkage preference helps to reduce the risk of avianhuman influenza infection. However, such transfers are possible. The 1918 pandemic influenza virus contained a mutation that was able to attach to both α(2→3) and α(2→6) sialic acids. In addition to sialic acid binding preferences, viral hemagglutinin binding may also have additional oligosaccharide structural requirements.23
After infection, the neuraminidase activity of the virus cleaves the sialic acid groups that hold the virus attached to the cell, releasing the newly formed viral particles. Two key antiviral drugs, zanamivir and oseltamivir, are neuraminidase inhibitors (NAI) developed using rational drug design and the protein structure of influenza neuraminidase.24 The mechanism of action of these inhibitors is to occupy the neuraminidase active site and block the glycolytic activity. The hemagglutinin activity of the newly formed virus particles attaches the particles to the host cell surface and prevents their escape into the extracellular space.25
In addition to neuraminidase inhibition as a means to combat influenza infection, sialic acid-based oligosaccharides have been screened for suitability as ligands for the influenza hemagglutinin. Theoretically, drugs based on sialyloglycoconjugates could be effective to prevent viral attachment to cell surfaces by competitively binding to the viral hemagglutinin.22
While the sialic acid binding abilities of viruses are under intensive study, other pathogenic bacteria, including Escherichia coli, Neisseria meningitidis, and Streptococcus agalactiae are able to synthesize N-acetylneuraminic acid. It has been hypothesized that these bacterial cell surface sialic acids mimic sialylated glycans on the host cell and camouflage the bacteria from the host immune system.26 A key enzyme in sialic acid synthesis, CMP sialic acid synthetase, has been identified as a potential target for development of drug therapies against bacterial pathogens.27
The parasite Plasmodium falciparum, which is the cause of malignant malaria in humans, uses hemagglutinins that preferentially bind to Neu5Ac to attach to human erythrocytes. However, P. falciparum has additional redundant infectious mechanisms that are sialic acid independent, contributing to the difficulty in developing preventative therapies for malaria.28
The depth of knowledge regarding sialic acids and the proteins that specifically bind to them has expanded rapidly; as of 2002 the number of sialic acid structures was thought to be around 40; another 10 structures have been identified in the subsequent decade. However, details of the processes that sialic acids participate in, the interpretations of those activities and why sialic acid expression changes occur in pathological states are still ambiguous and a focus of continuing research. Because changes in cellular sialylation have been associated with cancer, infection, diabetes, and other illnesses, sialic acid research is poised to be of interest to scientists who seek to understand the role of sialylation as a possible cause, effect, or indicator of these disorders.
Find more technical resources on our Glycobiology hub page.
Sialic Acids and Sialyl Oligosaccharides
Sialic Acid Synthesis
Sialic Acid Transferases
Sialic Acid Degradation Enzymes
Sialylated Polysaccharides
Desialylated Polysaccharides
Neuraminidases (Sialidases)
Neuraminidase Detection Substrates
Enzyme Inhibitors
Antibodies to Sialic Acid Metabolic Enzymes
Sialyl-binding Lectins
Sialic Acid Detection
References
1-20
21-28
如要继续阅读,请登录或创建帐户。
暂无帐户?